Research Topics
1) Bacterial adaptation in response to repeated resource limitation.
​
Microbes are robust organisms capable of persisting in the face of extreme environments. In nature, access to nutrients is not always consistent and populations commonly experience cycles of feast and famine. To further understand how microbes persist and eventually thrive in environments where nutrient availability is sporadic, we are performing evolution experiments varying intervals between feeding times in Escherichia coli populations. We will then characterize these lines to understand how microbes adapt to starvation and how these adaptations are constrained by the need to maintain fitness when resources are replenished.
Current questions include:
1) How does evolutionary history affects future adaptive trajectories?
(How does previous exposure to a specific interval of feast/famine affect evolution when the environment changes)
2) Is evolution predictable across a gradient of feast/famine intervals?
​
3) How can mutations that arise during experimental evolution increase our understanding of basic microbial physiology?
A
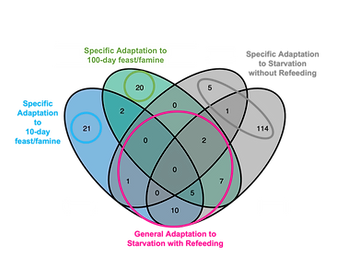

B
C


D
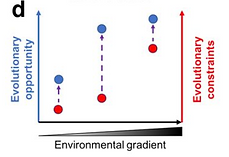
E
Evolution in response to feast/famine is specific to the duration and severity of starvation. Examples here include: A) Fixed mutations commonly arise in different loci depending on the duration and severity of starvation before refeeding. Venn diagram illustrates the number of distinct genes that are overrepresented for mutations following 900-days of feast/famine cycles (10-day [blue], 100-day [green]) or 1000-days of starvation without refeeding [grey]. Mutations that occur both during feast/famine and starvation without refeeding (circled in pink) may represent globally beneficial mutations for starvation.
B) Evolution across a gradient of feast/famine intervals has very different effects on the base-genetic mutation rate. Clones isolated from populations that evolved to 10-day feast famine cycles [pink] exhibit significantly increased mutation rates while clones from 100-day feast/famine cycles [green] exhibit reduced mutation rates. C) Evolution across a gradient also has very different effects on colony size. E. coli populations that evolved to 10-day feast/famine cycles produce extremely small colonies compared to populations that evolved in the other feast/famine conditions (evolved colonies are visualized in red). D) A possible explanation for why colonies from populations that evolved to 10-day feast famine cycles are so small may be due to the evolution of cooperative cross-feeding behaviors. Here, the growth of colonies is reduced without help from their cross-feeding partners. Transcriptomics reveals patterns of complementary gene expression between ecotypes that evolved in a 10-day feast/famine population as Ecotype A [red] invests less in fatty acid biosynthesis (top) and Ecotype B [blue] invests less in the scavenging of metals (bottom). Due to the cost of fatty-acid biosynthesis and metal scavenging, these processes are ideal targets for the evolution of cooperation by gene loss as described by the Black Queen Hypothesis. E) Evolutionary patterns observed across the gradient of feast/famine cycles are non-monotonic and may be related to economic theories of cost/benefit analysis. Here, populations may experience an increase in evolutionary opportunity as resource limitation becomes more severe (e.g., increases in beneficial mutation rates, distance from fitness optima) but, they also experience increases in evolutionary constraints (e.g., increases in deleterious mutation rates, decreases in effective population sizes, decreased resource availability, and fewer accessible mutational trajectories). If both the total evolutionary opportunity and evolutionary constraint increase such that their curves diverge and then re-approach, then the greatest net evolutionary opportunity can exist in an intermediate environment. These results illuminate the complexities in developing a framework for evolutionary predictability and why linear models may be inappropriate for interpolating the evolution of traits across stressful environmental gradients. Behringer and Ho, et al., bioRxiv ; Behringer et al, 2022, mBio ; Wei et al, 2022 Nature Communications
2) Effects of genotype, environment, and microbial life history on genetic and epigenetic mutation rates.
​
Microbes exhibit some of the lowest spontaneous mutation rates across the Tree of Life. Powered by low mutation rates and extremely large population sizes, microbes can efficiently sample new alleles and rapidly adapt to environmental changes while minimizing the burden of genetic load. However, because microbes have colonized every habitable niche on Earth, many species have evolved unique adaptations that make them difficult or impossible to culture in standard laboratory conditions and have been overlooked in studies of microbial mutation rates. Moreover, many microbes, particularly opportunistic pathogens, have life histories consisting of very different environmental conditions, which may result in mutation rate plasticity and greatly affect the molecular clock. As such, we are very interested in cataloging mutation rates in such organisms (obligate anaerobes, fastidious species) and determining the molecular and physiological features of these organisms that contribute to mutation/fidelity of DNA replication.
In addition to genetic mutations, microbes also maintain a variety of nucleotide base modifications across their genomes. This epigenetic information can be gained or lost, resulting in semi-permanent heritable change. Recent work by our lab (Stone et al, 2023, in press) has shown that the pattern of 6-methyladenine (6mA) modifications across the E. coli genome can evolve differently depending on genotype. In E. coli, the primary function of 6mA is to direct the mismatch repair (MMR) machinery and aid in the correction of DNA replication errors. In the absence of MMR, we found that the selective pressure maintaining 6mA methylation is relaxed and that genome-wide methylation erodes in a non-deterministic fashion. Future investigation by our lab will focus on estimates of 6mA epimutation rate and how the environment affects epimutation rates.
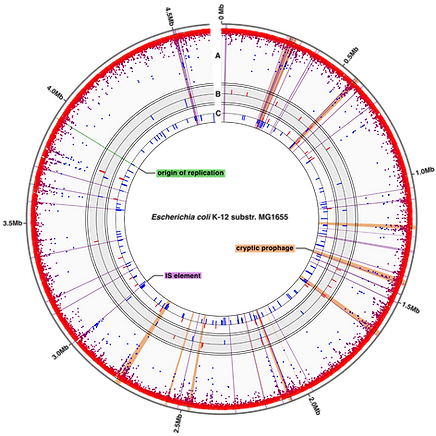
Genome-wide 6mA methylation in E. coli K-12 substr. MG1655. The origin of replication (green), IS elements (purple) and cryptic prophages (orange) are shown for reference. (A) Percent methylation at GATC sites. Each point represents one GATC site and points are colored to reflect percent methylation from 0% (blue, inside) to 100% (red, outside). (B-C) Differentially methylated GATC sites in evolved WT (A) and MMR- (B) clones. Red bars are sites with increased methylation and blue bars are decreased methylation, and the height of the bar reflects the difference in percent methylation between the ancestor and the evolved clones. Each ring ranges from -25% to 25%.
3) Genetic and environmental drivers of stable subpopulation structure in experimentally evolving Escherichia coli populations.
​
When provided with a rich array of nutrients and an opportunity for spatial segregation, microbes can take advantage of newly acquired genetic variation and rapidly diversify to occupy new unique niches. In a long-term evolution experiment, we have been evolving populations of E. coli in spatially complex (culture tubes) and spatially homogeneous (flasks) environments containing a nutritionally complex media (LB broth). In spatially complex environments, the first visual phenotypic change we observe is the presence of a biofilm at the surface/air interface of the culture tube. Whole-population shotgun genomic sequencing revealed that these populations contained parallel mutations, particularly affecting genes that influence biofilm formation (fimE, regulator of the type-I fimbriae (FimA); hns, global regulator of environmental-change and stress-response genes; and acrB, a subunit of the MDR family efflux pump AcrAB/TolC). Deletion of fimA renders E. coli populations unable to evolve the ability to form biofilms. Without the ability to evolve increases in biofilm formation, adaptation is hindered in the culture tube environment but not in the flask environment. Future work will focus on why the ability to evolve increases in biofilm formation is so important in the culture tube environment.
F
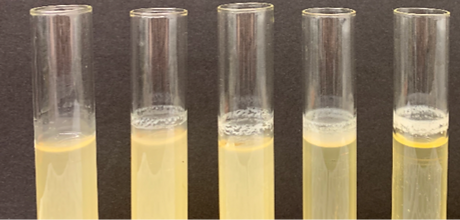
Ancestor
Evolved biofilm-forming clones
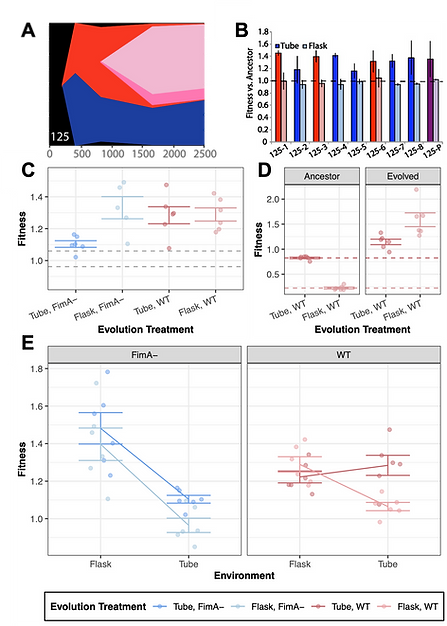
When cultivated in the presence of a rich, complex culture medium and in a culture vessel that provides the opportunity for spatial segregation, E. coli populations are observed to rapidly evolve stable subpopulation structure. A) Whole-population shotgun sequencing in combination with whole-genome shotgun sequencing of isolated clones reveals the presence of at least two independently-evolving ecotypes. In the context of population 125 (upper right) both a planktonic/bottom-dwelling subpopulation (red and pink area) and a biofilm-forming ecotype (blue area). B) Head to head competitions of evolved clones vs. the WT ancestor reveal that adaptation of both ecotypes is specific to the culture tube environment (dark bars) as no fitness gains are realized when competed in the more homogeneous flask environment (light bars). C) When evolution is replayed with a genetic background that is unable to form biofilms (FimA-, blue), adaptation is hindered in the culture tube environment but not the flask environment. D) FimA production in the WT ancestor is initially energetically wasteful as the WT ancestor has only a weak ability to produce biofilms, as such the WT ancestor is initially slightly less fit than the FimA- ancestor in culture tubes and significantly less fit in flasks (left panel). However, as WT lines evolve the become significantly more fit than FimA- ancestor in both environments (right panel).
E) Head to head competitions of evolved populations vs. their relative ancestor in both their "evolved" condition and their "reciprocal" condition reveals that WT populations evolve to be locally adapted (or specifically adapted to their evolved environment, left panel [red]). In contrast, FimA- populations always exhibit the greatest fitness in culture flasks regardless of their evolved environment. F) Image showing that the presence of biofilm in evolved populations is novel as the WT ancestor exhibits weak biofilm formation in the experimental culture environment. Patton et al, in prep. Behringer et al., 2018. PNAS.
4) Experimental and translational investigation of interactions between pathogenic and commensal microbes in the small intestine and urogenital microbiome. ​
​
It should also be noted that gene regulation by H-NS and the resulting expression of the type-I fimbriae are contributing factors to uropathogenic E. coli (UPEC) strains colonizing the bladder, resulting in urinary tract infection (UTI). As these genes are also implicated in the establishment and maintenance of subpopulation structure, it's possible that during infection the UPEC strains also evolve and diversify within the bladder - as they do in our long-term experiment - contributing to therapeutic failure in individuals with recurrent UTI. One obstacle that UPEC strains must overcome as they colonize the bladder is the presence of the native uroprotective microbiome which is comprised of a number of fastidious anaerobic microbes namely, Lactobacillus. One species, Lactobacillus crispatus, has been suggested to have an antagonistic relationship with E. coli and can exclude the growth of UPEC strains. Other Lactobacillus species similarly interact with E. coli in the gut microbiome, a known reservoir for urinary tract infection. Current and future work will focus on: characterizing how mutation rates fuel the evolution of Lactic Acid Bacteria, identifying strategies employed by UPEC strains to overcome Lactobacillus, and applying this knowledge to clinical E. coli isolates.